Maps of Fundamental Soil Layers
Maps show a range of soil attributes derived from the dominant soils depicted in the 1:50,000 scale NZ Fundamental Soil Layers.
Interpreting the maps
Most of the maps show the most likely (i.e. modal) value from the classed soil attribute data. Even within small areas of the same soil, soil attributes can be very variable. You can click on a soil area to see our estimate of the range of values that might be expected for each soil. Since it is quite impractical to measure soil attributes for all soils, we also provide a guide to the technique we used to derive our estimate. These range from 'measured', indicating the the mapped soil has been measured, to 'general inference', indicating the soil measured was generally similar to the mapped soil, but we have no actual measurements to support the inference.
Soil Attribute Maps
The dominant soil of a land resource inventory area is mapped according to the following key soil chemical attributes: minimum pH, maximum salinity, cation exchange capacity, total carbon, phosphate retention.
Minimum pH
Minimum pH classes and their corresponding values are described in relation to plant growth at 0.2–0.6 m depth. pH is an important soil attribute that significantly influences nutrient availability and aluminium toxicity. The pH classes originate from Parfitt (1984), and are as defined in Webb and Wilson (1995).
Maximum Salinity
Maximum salinity in the 0.0–0.6 m zone as percent soluble salts (g/100 g soil). Salinity negatively impacts plant growth, water quality and infrastructure. Salinity classes are as defined in Webb and Wilson (1995) and Milne et al. (1995).
Cation Exchange Capacity
Average Cation Exchange Capacity (CEC) at 0–0.6 m as centimoles of charge per kg (cmoles (+)/kg). CEC is an indicator of the capacity of a soil to hold nutrients and is an important component in effluent absorption and buffering capacity. CEC classes are as defined in Webb and Wilson (1995) and Blakemore et al. (1987).
Total Carbon
Total Carbon (%) as a weighted average for 0–0.2 m depth. Carbon content and, more specifically, organic matter are important drivers of soil characteristics such as nutrient attenuation, and soil aggregate stability, and may be reasonable indicators of soil health. The classes are defined in Webb and Wilson (1995) and Blakemore et al. (1987).
Phosphate Retention
Phosphate retention or anion storage capacity (%) as a weighted average for 0–0.2 m depth. Phosphate retention influences phosphate fertiliser requirements and soil structural stability. The classes are as defined in Blakemore et al. (1987) and Webb and Wilson (1995).
The dominant soil of a land resource inventory area is mapped according to the following key soil physical characteristics: topsoil gravel content, rock outcrops and surface boulders, and particle size.
Topsoil Gravel Content
The gravel content (%) of the top 20 cm of the soil profile (0–20cm). The amount of stones affects a number of hydraulic characteristics of the soil as well as its workability and root penetration characteristics. The classes are as described in Webb and Wilson (1995).
Rock outcrops and surface boulders
The coverage of rock outcrop and surface boulders (%). Rock outcrop and surface boulders act as a hindrance to machinery and constrain management practices. The classes are as defined in Webb and Wilson (1995)
Particle Size
Particle-size class describes the proportions of sand, silt, and clay in the fine earth fraction of the soil except, in the case of skeletal soils ( >35% coarse fraction) where it applies to the whole soil. Particle size is important for soil trafficability, soil workability, and moisture storage capacity and permeability. The classes are as described in Webb and Wilson (1995).
The dominant soil of a land resource inventory area is mapped according to the following key soil drainage parameters relevant to plant growth: potential rooting depth, depth to a slowly permeable horizon, internal soil drainage, and soil permeability.
Potential rooting depth
Potential rooting depth describes the depth (in metres) to a layer that may impede root extension. Such a layer may be defined by penetration resistance, poor aeration or very low available water capacity. Potential rooting depth is important for plant growth and soil workability. The classes are as defined in Webb and Wilson (1995).
Soil Permeability
Soil Permeability is described as a class. Profile permeability classes are determined in the soil profile below the A horizon by measuring hydraulic conductivity or by the field measurement methods of Griffiths (1985, 1991). Soil permeability, impacts drainage, water logging, effluent absorption potential, leaching, and water loss. Classes are as defined in Webb and Wilson (1995).
Depth to a Slowly Permeable Horizon
Depth to a slowly permeable horizon (meters). This is defined as an horizon in which the permeability is less than 4 mm/hr. If no slowly permeable horizon is observed, the soil is allocated a null value. Permeability is important for ease of drainage, risk of water logging, effluent absorption potential, leaching, and water loss. These classes are as defined in Webb and Wilson (1995) and the measurement methods in Griffiths (1985).
Soil Drainage
Soil drainage described as a class. Drainage classes are assessed either using criteria of soil depth and chroma, or from reference to diagnostic horizons. Soil drainage is important for the supply of oxygen to the plant root zone, waterlogging, and water drainage. Drainage classes used here are the same as those used in the NZ Soil Classification (Hewitt 1993), and as defined in Milne et al. (1995).
The dominant soil of a land resource inventory area is mapped according to the following key soil physical attributes: flood return interval and soil temperature regime (0.3 m depth).
Flood return interval
The classes are described more fully in Webb and Wilson (1995). Frequency of flooding is important for most land activites.
Soil temperature regime
Soil temperature (ºC)at 0.3 m depth. Soil temperature affects crop suitability and yield. The classes used originate from and are described more fully in Webb and Wilson (1995), which in turn is based on the work of Aldridge (1982, 1984) and Aldridge and Cook (1983).
The dominant soil of a land resource inventory area is mapped according to the following key soil physical attributes: profile total available water, profile readily available water, macroporosity 0–0.6 m depth and macroporosity 0.6–0.9 m depth.
Profile Total Available Water
Profile total available water (mm) for the soil profile to a depth of 0.9 m, or to the potential rooting depth (whichever is the lesser). Values are weighted averages over the specified profile section (0–0.9 m) and are expressed in units of mm of water. Profile total available water is important for droughtiness and overall water availability. The classes originate from the work of Gradwell and Birrell (1979), Wilson and Giltrap (1982), and Griffiths (1985), and are described more fully in Webb and Wilson (1995).
Profile Readily Available Water
Profile readily available water for the soil profile to a depth of 0.9 m, or to the potential rooting depth (whichever is the lesser). Values are weighted averages over the specified profile section (0–0.9 m) and are expressed in units of mm of water. Profile total available water is important for droughtiness and plant available water. The classes originate from the work of Gradwell and Birrell (1979), Wilson and Giltrap (1982), and Griffiths (1985), and are described more fully in Webb and Wilson (1995).
Macroporosity (0–0.6 m)
Macroporosity is an expression of the air-filled porosity of the soil at ‘field capacity’. Values are minimum values over the specified profile section (0–0.6 m), and are expressed as a percentage of the soil volume. Macroporosity is important for the characterization of supply of oxygen to plant roots, waterlogging, and ease of drainage. The classes originate from the work of Gradwell (1960) and Gradwell and Birrell (1979), and are described more fully in Webb and Wilson (1995).
Macroporosity (0.6–0.9 m)
Macroporosity is an expression of the air-filled porosity of the soil at ‘field capacity’. Values are minimum values over the specified profile section (0.6–0.9 m), and are expressed as a percentage of the soil volume. Macroporosity is important for the supply of oxygen to plant roots, waterlogging and ease of drainage at depth in the soil profile. The classes originate from the work of Gradwell (1960) and Gradwell and Birrell (1979), and are described more fully in Webb and Wilson (1995).
Aldridge R 1982. The prediction of soil temperature in New Zealand and application to temperature regimes of Soil Taxonomy. New Zealand Soil Bureau Scientific Report 54. 23p.
Aldridge R, Cook FJ 1983. Estimation of soil temperatures at 0.1 m and 0.3 m depths. New Zealand Soil Bureau Scientific Report 62. 18p.
Aldridge R 1984. Proposal for New Zealand soil temperature regimes. New Zealand Soil Bureau Soil Resources Report SR4. 12p.
Blakemore LC, Searle PL, Daly BK 1987. Methods for chemical analysis of soils. New Zealand Soil Bureau Scientific Report 80. 103p.
Gradwell MW 1960. Changes in the pore space of a pasture topsoil under animal treading. New Zealand Journal of Agricultural Research 3: 663–674.
Gradwell MW, Birrell KS 1979. Soil Bureau laboratory methods. Part C. Methods for physical analysis of soils. New Zealand Soil Bureau Scientific Report 10C.
Griffiths E 1985. Interpretation of soil morphology for assessing moisture movement and storage. New Zealand Soil Bureau Scientific Report 74. 20p.
Griffiths E 1991. Assessing permability class from soil morphology. DSIR Land Resources Technical Record 40. 48p.
Hewitt AE 1993. Methods and rationale of the New Zealand Soil Classification. Landcare Research Science Series 2. Lincoln, New Zealand, Manaaki Whenua Press. 71p.
Milne JDG, Clayden B, Singleton PL, Wilson AD 1995. Soil description handbook. Lincoln, New Zealand, Manaaki Whenua Press. 157p.
Parfitt RL 1984. Reserves of nutrients in New Zealand soils. New Zealand Soil News 32: 123–30.
Webb TH, Wilson AD 1995. A manual of land characteristics for evaluation of rural land. Landcare Research Science Series 10. Lincoln, New Zealand, Manaaki Whenua Press. 32p.
Wilson AD, Giltrap DJ 1982. Prediction and mapping of soil water retention properties. New Zealand Soil Bureau District Office Report WN7. 15p.
The New Zealand soil map based on NZLRI polygons
FSL New Zealand Soil Classification
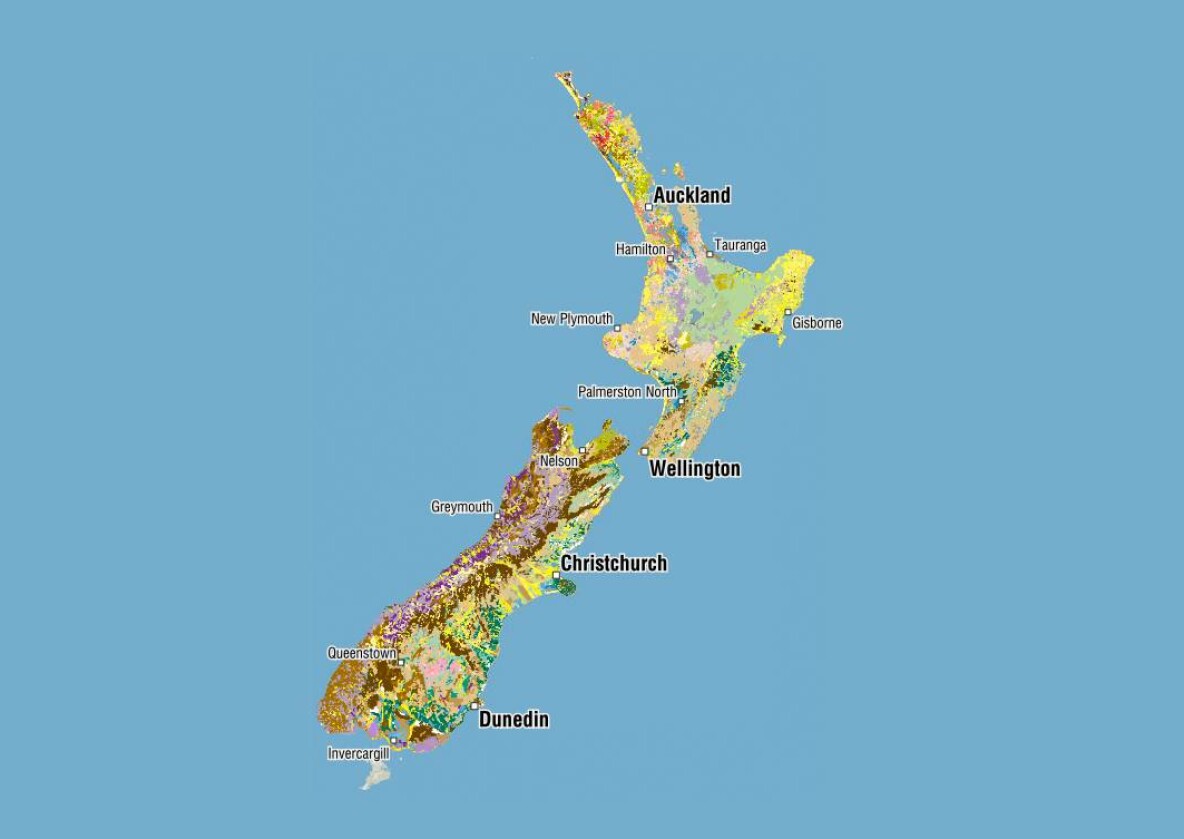